Factor to be considered for constructing a Generating Station.
During planning of construction of generating station the following factors to be considered for economical generation of electrical power.
1) Easy availability of water for thermal power generating station.
2) Easy availability of land for construction of power station including its staff township.
3) For hydro power station there must be a dam on river. So proper place on the river must be chosen in such a way that the construction of the dam can be done in most optimum way.
4) For thermal power station easy availability of fuel is one of the most important factors to be considered.
5) Better communication for goods as well as employees of the power station also to be kept into consideration.
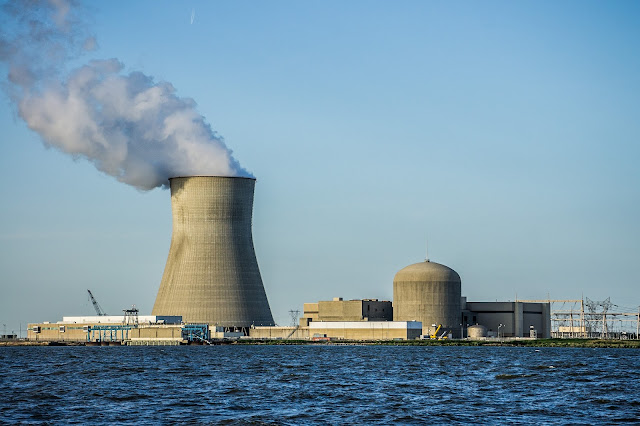 |
power plant |
6) For transporting very big spare parts of turbines, alternators etc., there must be wide road ways, rain communication, and deep and wide-river must pass away nearby the power station.
7) For nuclear power plant, it must be situated in such a distance from common location so that there may be any effect from nuclear reaction the heath of common people.
Many other factors also to be considered, but there are beyond the scope of our discussion. All the factors listed above are very difficult to be available at load center. The power station or generating station must be situated where all the facilities are easily available. This place may not be necessarily at the load center. The power generated at generating station then transmitted to the load center by means of electrical power transmission system as we said earlier. The power generated at generating station is in low voltage level as low voltage power generation has some economical values. Low voltage power generation is more economical than high voltage power generation. At low voltage level, bot weight and wide of insulation is less in the alternator, this directly reduces the cost and size of alternator. But this low voltage level power cannot be transmitted directly to the consumer end as because this low voltage power transmission is not at all economical. Hence although low voltage power generation is economical but low voltage electrical power transmission is not economical. Electrical power is directly proportional to the product of electrical current and voltage of system. So for transmitting certain electrical power from one place to another, if the voltage of the power is increased then associated current of this power is reduced. Reduced current means less I2R loss in the system, less cross sectional area of the conductor means less capital involvement and decreased current causes improvement in voltage regulation of power transmission system and improved voltage regulation indicates quality power. Because of these three reasons electrical power mainly transmitted at high voltage level. Again at distribution end for efficient distribution of the transmitted power, it is stepped down to its desired low voltage level. So it can be concluded that first the electrical power is generated at low voltage level then it stepped up to high voltage for efficient transmission of electrical energy. Lastly for distribution of electrical energy or power to different consumers it is stepped down to desired low voltage level. This brief discussion of electrical transmission system and network, but now we will discussed little bit more details about transmission of electrical energy.
Transmission
of Electrical Energy
Fundamentally
there are two systems by which electrical energy can be transmitted.
(1)
High voltage DC electrical transmission system.
(2)
High voltage AC electrical transmission system. There are some advantages in
using DC transmission system-
i)
Only two conductor are required for DC transmission system. It is further
possible to use only one conductor of DC transmission system if earth is
utilized as return path of the system.
ii)
The potential stress on the insulator of DC transmission system is about 70% of
same voltage AC transmission system. Hence less insulation cost is involved in
DC transmission system.
iii)
Inductance, capacitance, phase displacement and surge problems can be eliminated
in DC system.
Even
having these advantages in DC system, generally electrical energy is transmitted
by three (3) phase AC transmission system.
i)
The alternating voltages can easily be stepped up & down, which is not
possible in DC transmission system.
ii)
Maintenance of AC substation is quite easy and economical compared to DC system.
iii)
The transforming in AC electrical substation is much easier than motor-generator
sets in DC system.
But
AC transmission system also have some disadvantages like,
i)
The volume of conductor used in AC system is much higher than that of DC.
ii)
The reactance of the line, affects the voltage regulation of electrical power
transmission system.
iii)
Problems of skin effects and proximity effects only found in AC system.
iv)
AC transmission system is more likely to be affected by corona effect than DC
system.
v)
Construction of AC electrical power transmission network is more completed than
DC system.
vi)
Proper synchronizing is required before inter connecting two or more
transmission lines together, synchronizing can totally be omitted in DC
transmission system.
Short
Transmission Line
The
transmission lines which have length less than 80 km are generally referred as
short transmission lines. For short length, the shunt capacitance of this
type of line is neglected and other parameters like electrical resistance and
inductor of these short lines are lumped, hence the equivalent circuit is
represented as given below,
Let’s
draw the vector diagram for this equivalent circuit, taking receiving end
current Ir as reference. The sending end and receiving end voltages
make angle with that reference receiving end current, of φs and
φr, respectively.
 |
short transmission lines |
As the shunt capacitance of the line is neglected, hence sending end
current and receiving end current is same, i.e. Is = Ir.
Now if we observe the vector diagram carefully, we will get, Vs is
approximately equal to, Vr + Ir.R.cosφr + Ir.X.sinφr
That means, Vs ≅ Vr + Ir.R.cosφr + Ir.X.sinφr
as the it is assumed that φs ≅ φr As there is
no capacitance, during no load condition the current through the line is
considered as zero, hence at no load condition, receiving end voltage is the
same as sending end voltage. As per dentition of voltage regulation of power
transmission line,
As there is
no capacitance, during no load condition the current through the line is
considered as zero, hence at no load condition, receiving end voltage is the
same as sending end voltage. As per dentition of voltage regulation of power
transmission line, As there is no capacitance, during no load condition the current
through the line is considered as zero, hence at no load condition, receiving
end voltage is the same as sending end voltage. As per dentition of voltage
regulation of power transmission line,As there is no capacitance, during no load condition the current
through the line is considered as zero, hence at no load condition, receiving
end voltage is the same as sending end voltage. As per dentition of voltage
regulation of power transmission line,
Here,
vr and vx are the per unit resistance and reactance of the
short transmission line.
Any
electrical network generally has two input terminals and two output terminals.
If we consider any complex electrical network in a black box, it will have two
input terminals and output terminals. This network is called two - port network.
Two port model of a network simplifies the network solving technique.
Mathematically a two port network can be solved by 2 by 2 matrix. A transmission
as it is also an electrical network, line can be represented as two port
network. Hence two port network of transmission line can be represented as 2 by
2 matrixes. Here the concept of ABCD parameters comes. Voltage and currents of
the network can represented as,
Where
A, B, C and D are different constant of the network. If we put Ir = 0
at equation (1), we get,
Hence,
A is the voltage impressed at the sending end per volt at the receiving end when
receiving end is open. It is dimension less. If we put Vr = 0 at
equation (1), we get
That
indicates it is impedance of the transmission line when the receiving terminals
are short circuited. This parameter is referred as transfer impedance.
C
is the current in amperes into the sending end per volt on open circuited
receiving end. It has the dimension of admittance.
D
is the current in amperes into the sending end per amp on short circuited
receiving end. It is dimensionless. Now from equivalent circuit, it is found
that, Vs = Vr + IrZ and Is =
Ir
Comparing these equations with equation 1 and 2 we get, A = 1,
B = Z, C = 0 and D = 1. As we know that the constant A, B, C and D are related
for passive network as,
AD
− BC = 1.
Here,
A = 1, B = Z, C = 0 and D = 1
⇒
1.1 − Z.0 = 1
So
the values calculated are correct for short transmission line. From above
equation (1),
When
Ir = 0 that means receiving end terminals is open circuited and then
from the equation 1, we get receiving end voltage at no
load.
and
as per definition of voltage regulation of power transmission
line,
Efficiency
of Short Transmission Line
The
efficiency of short line as simple as efficiency equation of any other
electrical equipment, that means
R is per phase electrical resistance of the transmission
line.
Medium
Transmission Line
The
transmission line having its effective length more than 80 km but less than 250
km, is generally referred to as a medium transmission line. Due to the
line length being considerably high, admittance Y of the network does play a
role in calculating the effective circuit parameters, unlike in the case of
short transmission lines. For this reason the modelling of a medium length
transmission line is done using lumped shunt admittance along with the
lumped impedance in series to the circuit.
These
lumped parameters of a medium length transmission line can be represented using
two different models, namely- 1).Nominal Π representation. 2).Nominal
T representation. Let’s now go into the detailed discussion of these
above mentioned models.
Nominal
Π Representation of a Medium Transmission Line
In
case of a nominal Π representation, the lumped series impedance is placed
at the middle of the circuit where as the shunt admittances are at the ends. As
we can see from the diagram of the Π network below, the total lumped shunt
admittance is divided into 2 equal halves, and each half with value Y ⁄ 2 is
placed at both the sending and the receiving end while the entire circuit
impedance is between the two. The shape of the circuit so formed resembles that
of a symbol Π, and for this reason it is known as the nominal Π
representation of a medium transmission line. It is mainly used for determining
the general circuit parameters and performing load flow
analysis.
As
we can see here, VS and VR is the supply and receiving end
voltages respectively, and Is is the current flowing through the
supply end. IR is the current flowing through the receiving end of
the circuit. I1 and I3 are the values of currents flowing
through the admittances. And I2 is the current through the impedance
Z. Now applying KCL, at node P, we get.
Similarly
applying KCL, to node Q.
Now
substituting equation (2) to equation (1)
Now
by applying KVL to the circuit,
Comparing
equation (4) and (5) with the standard ABCD parameter
equations
We
derive the parameters of a medium transmission line as:
Nominal
T Representation of a Medium Transmission Line
In
the nominal T model of a medium transmission line the lumped shunt
admittance is placed in the middle, while the net series impedance is divided
into two equal halves and and placed on either side of the shunt admittance. The
circuit so formed resembles the symbol of a capital T, and hence is known
as the nominal T network of a medium length transmission line and is shown in
the diagram below.
Here
also Vs and Vr is the supply and receiving end voltages
respectively, and Is is the current flowing through the supply end.
Ir is the current flowing through the receiving end of the
circuit.
Let
M be a node at the midpoint of the circuit, and the drop at M, be given by
Vm. Applying KVL to the above network we get,
Now
the sending end current is,
Substituting
the value of VM to equation (9) we get,
Again
comparing equation (8) and (10) with the standard ABCD parameter
equations,
The
parameters of the T network of a medium transmission line are
Long
Transmission Line
A
power transmission line with its effective length of around 250 Kms or above is
referred to as a long transmission line. Calculations related to circuit
parameters (ABCD parameters) of such a power transmission is not that simple, as
was the case for a short transmission line or medium transmission
line.
The
reason being that, the effective circuit length in this case is much higher than
what it was for the former models (long and medium line) and, thus ruling out
the approximations considered there like.
a)
Ignoring the shunt admittance of the network, like in a small transmission line
model.
b)
Considering the circuit impedance and admittance to be lumped and concentrated
at a point as was the case for the medium line model. Rather, for all practical
reasons we should consider the circuit impedance and admittance to be
distributed over the entire circuit length as shown in the figure below. The
calculations of circuit parameters for this reason is going to be slightly more
rigorous as we will see here. For accurate modelling to determine circuit
parameters let us consider the circuit of the long transmission line as
shown in the diagram below.
Here
a line of length l > 250km is supplied with a sending end voltage and current
of VS and IS respectively, where as the VR and
IR are the values of voltage and current obtained from the receiving
end. Let’s us now consider an element of infinitely small length Δx at a
distance x from the receiving end as shown in the figure
where,
V
= value of voltage just before entering the element Δx. I = value of current
just before entering the element Δx. V+ΔV = voltage leaving the element Δx. I+ΔI
= current leaving the element Δx. ΔV = voltage drop across element Δx. zΔx =
series impedence of element Δx yΔx = shunt admittance of element Δx Where Z = z
l and Y = y l are the values of total impedance and admittance of the long
transmission line. Therefore, the voltage drop across the infinitely small
element Δx is given by,
Now
to determine the current ΔI, we apply KCL to node A. ΔI = (V+ΔV)yΔx = V yΔx + ΔV
yΔx Since the term ΔV yΔx is the product of 2 infinitely small values, we can
ignore it for the sake of easier calculation. Therefore, we can write dI ⁄ dx =
V y ----------------- (2)
Now
by derivation both sides of eq (1) w.r.t x, d2 V ⁄ d x2 =
z dI ⁄ dx
Now
substituting dI ⁄ dx = V y from equation (2) d2 V ⁄ d x2 =
zyV or d2 V ⁄ d x2 − zyV = 0 ------------
(3)
The
solution of the above second order differential equation is given by. V =
A1 ex√yz + A2 e−x√yz --------------
(4)
Derivation
equation (4) w.r.to x. dV/dx = √(yz) A1 ex√yz −
√(yz)A2 e−x√yz ------------(5)
Now
comparing equation (1) with equation (5)
Now
to go further let us define the characteristic impedance Zc and
propagation constant δ of a long transmission line as
Zc
= √(z/y) Ω
δ
= √(yz)
Then
the voltage and current equation can be expressed in terms of characteristic
impedance and propagation constant as
V
= A1 eδx + A2 e−δx -----------
(7)
I
= A1/ Zc eδx + A2 / Zc
e−δx --------------- (8)
Now
at x=0, V= VR and I= Ir. Substituting these conditions to
equation (7) and (8) respectively.
VR
= A1 + A2 --------------- (9)
IR
= A1/ Zc + A2 / Zc ---------------
(10)
Solving
equation (9) and (10), we get values of A1 and A2
as,
A1
= (VR + ZCIR) ⁄ 2
And
A1 = (VR − ZCIR) ⁄
2
Now
applying another extreme condition at x=l, we have V = VS and I =
IS.
Now
to determine VS and IS we substitute x by l and put the
values of A1 and A2 in equation (7) and (8) we
get
VS
= (VR + ZC IR)eδl ⁄ 2 +
(VR − ZC IR)e−δl/2
--------------(11)
IS
= (VR ⁄ ZC + IR)eδl/2 −
(VR / ZC −
IR)e−δl/2--------------- (12)
By
trigonometric and exponential operators we know
sinh
δl = (eδl − e−δl) ⁄ 2
And
cosh δl = (eδl + e−δl) ⁄ 2
Therefore,
equation(11) and (12) can be re-written as
VS
= VRcosh δl + ZC IR sinh
δl
IS
= (VR sinh δl)/ZC + IRcosh
δl
Thus
comparing with the general circuit parameters equation, we get the ABCD
parameters of a long transmission line as,
A
= cosh δl
B
= ZC sinh δl
C
= sinh δl ⁄ ZC
D
= cosh δl
ABCD
Parameters of Transmission Line
A major section of power system engineering deals in the
transmission of electrical power from one particular place (e.g. generating
station) to another like substations or distribution units with maximum
efficiency. So it’s of substantial importance for power system engineers to be
thorough with its mathematical modelling. Thus the entire transmission system
can be simplified to a two
port network for the sake of easier calculations.
The circuit of a 2 port network is shown in the diagram below. As the name
suggests, a 2 port network consists of an input port PQ and an output port RS.
Each port has 2 terminals to connect itself to the external circuit. Thus it is
essentially a 2 port or a 4 terminal circuit, having,
Given to the input port P Q.
Given to the output port R S. As shown in the diagram below. Now the
ABCD
parameters or the transmission line parameters provide the link
between the supply and receiving end voltages and currents, considering the
circuit elements to be linear in nature. Thus the relation between the sending
and receiving end specifications are given using
ABCD
parameters by the equations below.
Now in order to determine the ABCD parameters of transmission line let us
impose the required circuit conditions in different cases.
ABCD Parameters,
When Receiving End is Open Circuited
The receiving end is open circuited meaning receiving end
current I
R = 0. Applying this condition to equation (1) we get,
Thus it implies that on applying open circuit condition to
ABCD parameters, we get parameter A as the ratio of sending end voltage to the
open circuit receiving end voltage. Since dimension wise A is a ratio of voltage
to voltage, A is a dimension less parameter. Applying the same open circuit
condition i.e I
R = 0 to equation (2)
Thus its implies that on applying open circuit condition to
ABCD parameters of transmission line, we get parameter C as the ratio of sending
end current to the open circuit receiving end voltage. Since dimension wise C is
a ratio of current to voltage, its unit is mho. Thus C is the open circuit
conductance and is given by C = IS ⁄ VR mho.
ABCD Parameters,
When Receiving End is Short Circuited
Receiving end is short circuited meaning receiving end
voltage V
R = 0 Applying this condition to equation (1) we get,
Thus its implies that on applying short circuit condition to
ABCD parameters, we get parameter B as the ratio of sending end voltage to the
short circuit receiving end current. Since dimension wise B is a ratio of
voltage to current, its unit is Ω. Thus B is the short circuit resistance and is
given by B = VS ⁄ IR Ω. Applying the same short circuit
condition i.e VR = 0 to equation (2) we get
Thus its implies that on applying short circuit condition to
ABCD parameters, we get parameter D as the ratio of sending end current to the
short circuit receiving end current. Since dimension wise D is a ratio of
current to current, it’s a dimension less parameter.
∴
The ABCD parameters of transmission line can be tabulated as:-
Parameter
|
Specification
|
Unit
|
A = VS / VR
|
Voltage ratio
|
Unit less
|
B = VS / IR
|
Short circuit resistance
|
Ω
|
C = IS / VR
|
Open circuit conductance
|
mho
|
D = IS / IR
|
Current ratio
|
Unit less
|
Performance
of Transmission Line
The
transmission lines are categorized as three types-
1)
Short transmission line– the line length is up to 80 km.
2)
Medium transmission line– the line length is between 80km to 160 km.
3)
Long transmission line – the line length is more than 160 km.
Whatever
may be the category of transmission line, the main aim is to transmit power from
one end to another. Like other electrical system, the transmission network also
will have some power loss and voltage drop during transmitting power from
sending end to receiving end. Hence, performance of transmission line can be
determined by its efficiency and voltage regulation.
Voltage
regulation of transmission line is measure of change of receiving end voltage
from no-load to full load condition.
Every
transmission line will have three basic electrical parameters. The conductors of
the line will have electrical resistance, inductance, and capacitance. As the
transmission line is a set of conductors being run from one place to another
supported by transmission towers, the parameters are distributed uniformly along
the line. The electrical power is transmitted over a transmission line with a
speed of light that is 3X108 m ⁄ sec. Frequency of the power is 50
Hz. The wave length of the voltage and current of the power can be determined by
the equation given below, f.λ = v where f is power frequency, λ is wave length
and υ is the speed of light.
Hence
the wave length of the transmitting power is quite long compared to the
generally used line length of transmission line.
For
this reason, the transmission line, with length less than 160 km, the parameters
are assumed to be lumped and not distributed. Such lines are known as
electrically short transmission line. This electrically short transmission lines
are again categorized as short transmission line (length up to 80 km) and medium
transmission line (length between 80 and 160 km). The capacitive parameter of
short transmission line is ignored whereas in case of medium length line the
capacitance is assumed to be lumped at the middle of the line or half of the
capacitance may be considered to be lumped at each ends of the transmission
line. Lines with length more than 160 km, the parameters are considered to be
distributed over the line. This is called long transmission line.
Voltage
in Power Electric Lines
In generating station electrical power is generated at medium
voltage level that ranges from 11 kV to 25 kV. This generated power is sent to
the generating step up transformer to make the voltage level higher. From this
point to the user end voltage level varies in different levels. We can realize
this voltage level variation step by step.
- At 11 kV or more than that up to 25 kV voltage level is
maintained at alternator stator terminals to generate electrical power in the
generating station.
- This generated power is fed to the generating step up
transformer to make this medium voltage level to higher level, i.e. up to 33 kV.
- Power at 33 kV is sent to the generating substation. There the
transformer increases the voltage level to 66 kV or 132 kV.
- From this generating substation power is sent to the nearer
substation to increase the voltage level higher than previous. This level of
voltage is increased at different suitable levels, it may be at 400 kV or 765 kV
or 1000 kV. This high voltage or extra high voltage level is maintained to
transmit the power to a long distant substation. It is call primary transmission
of power.
- At the end point of primary transmission of power, in the
substation, the step down transformers are used to step down the voltage level
to 132 kV. Secondary transmission of power starts from this substation.
- Power transformer at the end of the secondary transmission, just
makes 132 kV voltage level step down to 33 kv or 11 kV as per requirement. From
this point, primary distribution of power starts to distribute power to
different distribution stations.
- At the end of primary distribution, the distribution stations
receive this power and step down this voltage level of 11 kV or 33 kV to 415 V
(Line Voltage). From these distribution stations to consumer ends, 415 V is kept
to sustain for utilization purpose.
Type of Power
Lines
From the very beginning of power generation to the user end
transmission lines are broadly classified based on different voltage levels.
Why High Voltage
is used for Long Transmission Line?
Generally long distant transmission lines are designed to
operate at high voltage, extra high or ultra high voltage level. It is because
of line power loss reduction purpose. Practically long distant transmission line
resistance is comparatively more than medium and short transmission line. Due to
this higher valued transmission line resistance considerable amount of power is
lost. So we need to decrease the amount of current through each conductor by
making the operating voltage very high for same amount of power transmission. We
know that the power in AC system to transmit is,
Total power loss P
Loss =
3I
L2R considering three phases altogether. R is the
resistance in ohm per phase of the transmission line. Now, rearranging Equation
(1) we get,
Again in DC system, there is no phase difference between
voltage and current, i.e. cosƟ = 1, and only two conductors (positive and
negative) are used. So, in DC system transmitted power P = VI, and power
loss,
From equation (2) and (3), it is clear that power loss in
transmission line is inversely proportional to the square of line voltage. The
higher value of line voltage the lesser amount of power loss occurs. Hence
transmission line conductor is used with less diameter, hence savings of
conductor material.
Why HVAC is used
for Long Transmission Line?
Now a day’s electrical energy is generated, transmitted and
distributed in AC form. Especially for long distant transmission line High
Voltage AC is transmitted for several reasons, they are:
- AC voltage can be stepped up or down as per requirement easily
by transformer.
- Maintenance of AC substation is easy and cheaper.
- Throughout electrical power system AC voltage is handled. So no
extra hazard of rectification or inversion like DC voltage transmission.
Why HVDC is used
for Long Transmission Line?
High Voltage DC is used at extra or ultra-high voltage level.
HVDC transmission is used at fixed level of voltage in primary transmission only
as it cannot be stepped up or down by transformer. Only in long distant
transmission line it is used only, because
- Only two conductors (positive and negative) are required.
- Absence of inductance, capacitance and phase displacement power
loss is very less. Hence better voltage regulation.
- Surge problem never occurs.
- No skin effect.
- Less insulation requires due to less potential stress.
- Less corona effect, thus less power loss.
- Highly stabilized and synchronized.
Why Low and
Medium Voltage is Used in Distribution Line?
In primary distribution, power is handled at 11 kV or 33 kV.
As voltage level gets stepped down from 132 kV to 11 kV or 33 kV, current level
gets higher valued. But this high valued current distributed among various local
distribution stations (distribution transformers) nearby. These distribution
transformers again steps down the voltage to 415 V. It is because; Power at 415
V is used at the user end. Distance between these distribution transformers and
the primary distribution stations is very short, hence conductor resistance is
not large. Very small amount of power is lost in this section.
Disadvantages of
AC or HVAC Transmission
The main disadvantages of AC transmission are
- AC lines require more conductor material than DC.
- AC transmission line construction is more complicated than DC.
- Effective resistance is increased due to skin effect, hence
power loss.
- Continuous power loss due to charging current because of line
capacitance.
Disadvantages of
DC or HVDC Transmission
The main disadvantages of DC transmission are
- Electric power is not generated in HVDC form due to commutation
problem. Only HVDC is achieved for transmission from HVAC by rectification. So
special arrangement is required for this conversion.
- DC voltage cannot be stepped up or down for transmission.
- DC switches and circuit breakers are expensive and with certain
limitations.
High Voltage
Transmission Lines
Distribution in simple words means to make available a number
of product or services to end customers. Same is the case when we are talking
about electrical distribution system. Means making available that 415v or 240v
to a number of end consumers through a distribution system. The generation
normally takes place around 11 Kv to 25 Kv which is generally termed as medium
voltage. Since we all aware of the fact that this is not the proper voltage for
transmission. So this voltage is the stepped up to a level of 220 kv or 400 kv
or 132 kv depending on the power transmitted or we can say that depends upon the
distance. This power is carried away through a transmission network of high
voltage lines. These transmission lines are of hundreds of kilometres. These
lines are then terminated to some substations which step down the desired
voltage to either 11kv or 33 kv or 66 kv. This can be termed as sub transmission
network.
Now if we are particularly taking about distribution network then everything
below 33kv i.e 11 kv feeders emanates from 33 kv is branched into several 11 kv
feeders. The main motto of these 11 kv feeders is to carry power close to the
nearby localities which include industrial area, villages etc. here the
transformer further reduce the 11 kv to 415v or 240v and it provides the voltage
through 415v feeders which in turn gives 415v or 240 v. now 415v is a three
phase supply so this is given to the places where we have to give three phase
supply. Similarly you all are aware of 220 v supply, it’s a single phase and is
used in homes. Example of Simple distribution system Let it there are 4
generating unit generating a total of 20kv this is step up to 400kv and
transmitted to the distribution network as explained above The distributing
substation receive it as either 66/33/11Kv there may be chance that there are
separate 66kv feeders but at the end. Those 11 kv or 33 kv are directly given to
DISCOMS. So in short if i am saying distribution system then it starts from 33or
11 kv downwards this is step down to 430/250 v for the customers by considering
the fact that there is voltage drop in transmission lines
A simple example demonstrates this Input HT lines 11kv from X grid 11 kv from
Y grid transformer rated primary voltage 11kv secondary voltage 416/240 v For
domestic users a phase and a neutral connection is supplied and for heavy load 3
wire connection is supplied For the distribution to the consumer end, we can use
either overhead line or underground line. Underground one are good in appearance
and safe but they are costly one. A typical length of 11 kv feeder on city os
around 3 km. where as in rural areas this length is up to 15-18 kms. A 415
feeder normally is of 0.5m to 1 km max Ring Main Unit - RMU It is mainly used by
distributors when more than one feeder is supplying input and in case of failure
from any feeder, powers can be fed uninterruptedly from other feeders at same
point. Now a days it is of SF
6 type. It is used for two inputs or one
outgoing to the load or one input or two outgoing to the load.
Electricity
supply to consumers
The secondary winding of the transformer is mostly of wye connection type.
This supply system with a neutral wire is termed as 4 wire 3 phase supply.4 wire
supplies are normally used to distribute domestic supplies since they can
provide an earthed neutral. The three phase wires together give a 3-phase 3-wire
supply (240x1.73=415Volts) suitable or heavy machinery such as 3-phase
motors.
Issues in
distribution Power system
Lack of priority is given to 33kv substations related to load and maintenance
also the health status of 11KV/415V. Absence of monitoring, overloading, result
in the improper voltage distribution which also leads to the breakdown of
equipment at consumer end. We are currently using circuit breakers for load
management moreover we don't have switches in distribution network, in order to
isolate certain loads for load shedding purpose. We know have CB(circuit
breakers) for 11kv feeders. So when desired it will leads to the isolation of
that particular network.